Aging is a problem nearly as old as life itself. It's a problem that humans have failed to solve over and over again. Historically, attempts ranged from the adventurous quest for the fountain of youth by Ponce De Leon, to the ancient Egyptians practicing alchemy in search of immortality. More recently, attempts have involved the once-promising research into antioxidants and the over-hyped results from calorie restriction. The one common denominator: all of these have failed to meaningfully improve maximum lifespan. So with failures spanning over thousands of years, why should we be optimistic about the future of anti-aging?
The main reason is simple: we are at the cusp of the genetic revolution. There are fundamental discoveries happening at an ever increasing pace in the field of genetics and cell biology that will translate into meaningful, real-world improvements.
Genome Sequencing
The perfect example is genome sequencing. The Human Genome Project was a global effort to map out the human genome that started in 1990 and was completed in 2003. Not only did this take 13 years to complete, it cost an estimated $3 billion. Prices to sequence the human genome have fallen even faster than Moore's Law, which states that the number of transistors on a computer chip doubles every two years. Think about that: genetic sequencing has improved at a faster rate than computers! Today it is possible to go online and purchase a DNA testing kit for as low as $199 and get results in a month or two. Not a bad improvement since the technology was first introduced.
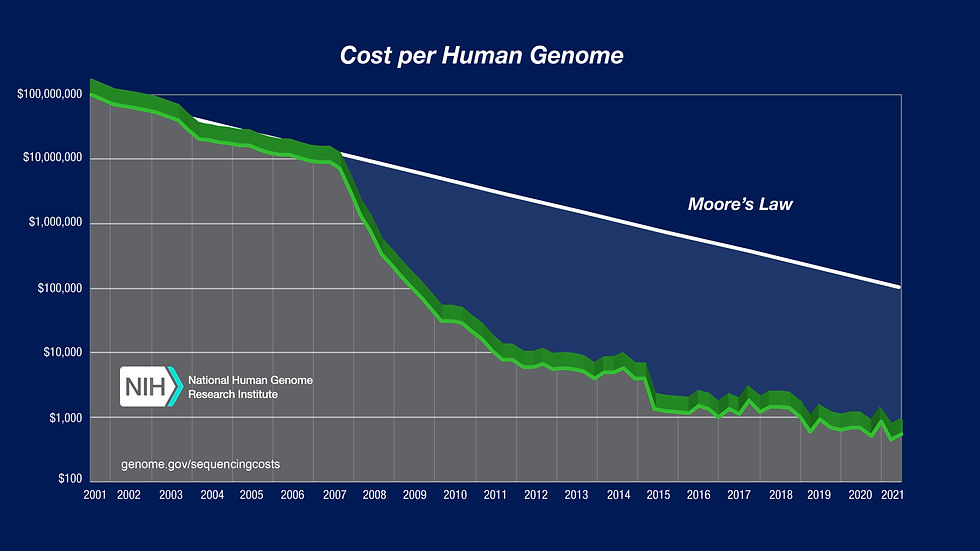
Cost of Sequencing a Human Genome
iPSCs
One of the least intuitive aspects of biology is that all of our cells have the same DNA, yet look wildly different. Your bones, skin, brain, heart, etc. all have the same DNA code. How is this possible? The answer is epigenetics. Epigenetics is an additional layer on top of the DNA that turns on/off specific genes in certain cell types. This is how our different cell types can express distinct genes while having the same master code.
At the beginning of human development, there is a single, remarkable cell known as a zygote. This single cell will give rise to all the different cell types in our body. The ability of the zygote to differentiate into different cell types is called pluripotency (meaning "several powers"). As cells become more specialized, they lose the power to divide into different cell types. This concept is often represented pictorially in Waddington's Epigenetic Landscape.
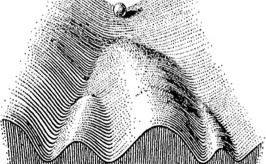
Waddington's Epigenetic Landscape
The ball in Waddington's epigenetic landscape analogy represents a developing cell, while the valleys represent distinct cell fates. As the ball (cell) rolls down the hill, it settles into a valley and cannot roll back up and move to a different valley. This concept mirrors the idea that once a cell has differentiated into a specific type, such as a skin cell, it cannot reverse its fate and become a different type of cell, such as a neuron. However, the question has to be asked: Is there some way to roll this ball back up the hill? Can we turn already differentiated cells into different cell types? The answer, surprisingly, is yes.
Japanese researcher and 2012 Nobel prize winner, Shinya Yamanaka, was able to transform a differentiated cell into a pluripotent cell by applying 4 factors, which have come to be known as the Yamanaka Factors. In terms of the analogy above, he found a way to roll the ball back up the hill. These cells became known as induced pluripotent stem cells, or iPSCs. iPSC's have unlocked an almost unlimited potential to regrow different tissues and open the door to regenerative medicine.
Cellular Reprogramming
We wouldn't want to take a pill or get an injection of the Yamanaka Factors, because that would result in our bodies producing teratomas (a tumor that contains many different kinds of body tissues). However, researchers have found that using 3 out of the 4 Yamanaka factors has the effect of rejuvenating cells, essentially rewinding their age to a more youthful state without altering their cell type. This process, called cellular reprogramming, is one of the hottest areas of research right now, and is very likely to result in a treatment to reduce the biological age of cells.
Epigenetic clocks
We covered earlier that the reason our cells are different is because certain genes are turned on/off in our cells through an additional layer called epigenetics. So what is epigenetics? Simplistically, there are two main mechanisms to how epigenetics work: methylation and acetylation. Small molecules are added and removed from DNA to serve as a signal to turn on/off a gene. As we age, these signals essentially become less clear, resulting in a loss of information. Professor and researcher, Steve Horvath, developed a model that shows we can accurately determine age by measuring these epigenetic signals on our DNA. This breakthrough gives researchers a way to measure aging, aside from looking at a birth certificate. Epigenetic clocks also gives scientists a mechanism to target. If epigenetic markers can be used to calculate age, are they potentially responsible for many of the effects of aging? We will see.
CRISPR/Base-Editing
The last fundamental technology for the genetic revolution is a big one: CRISPR. CRISPR, or Clustered Regularly Interspaced Short Palindromic Repeats, was derived from what can be essentially thought of as a bacteria's immune system. CRISPR was discovered by Emmanuelle Charpentier and Jennifer Doudna (two more Nobel prize winners in 2020). CRISPR can be used to identify a specific region of DNA and create a double stranded break. This can be useful to destroy, or more scientifically, knock down a gene that is causing a disease, or potentially replace a section of undesirable DNA with desirable DNA through genetic processes such as Homology Directed Repair or Non-Homologous End Joining. Multiple Companies have reached billion dollar valuations developing solutions to diseases such as A1 anti-trypsin disease, beta-thalassemia, sickle cell disease, familial hypercholesterolemia and more to come.
A further iteration, aka CRISPR 2.0, is already in the works called Base editing. Base editing is similar to CRISPR in that it uses a DNA binding domain to locate a very specific section of DNA and change the genetic code without causing a double stranded break in the DNA. Double stranded breaks have some undesirable health effects, so this iteration would likely be preferred for treatment in humans.
Bonus Technology: Artificial Intelligence
The understatement of the decade may be calling artificial intelligence just a bonus. From mastering chess in a few hours and becoming so strong it could beat all the most advanced engines, to being able to replicate a persons voice after listening to a few minutes of their audio, this technology is increasing exponentially. While there are hundreds of ways this is being applied, let's focus on one: the protein folding problem. Determining how a chain of amino acids folds into a protein has been an incredibly difficult problem to solve up to this point. Enter Alphafold. This neural network is the first program that can accurately predict the 3D structure of a protein without relying on experimental methods. This technology will be exceptionally useful when combined with the CRISPR/base-editing technology. Scientists will be able to create a gene for a new protein and predict its shape and function without the massive investment for many experiments.
Conclusion
Take a moment to imagine what the future will look like. You order a DNA kit online, spit into a tube, and have your DNA sequenced for a few hundred dollars to identify health risks decades before you experience symptoms. You have some skin cells scraped off in a painless procedure and they are turned into whatever cell type you need via iPSCs using the Yamanaka Factors. Any errors in your DNA can be corrected through Base-Editing or CRISPR, and the corrected cells can be put back into your body. Every decade, you go to the rejuvenation clinic for your cellular reprogramming to rejuvenate your existing cells that have started to get old. While this scenario may seem like something out of Sci-Fi, it will be here in the not so distant future.
These five technologies, plus a bonus, will lead us into the genetic revolution. All are relatively new, all are increasing exponentially, and all have the potential to make a great impact on improving healthspan and maximum lifespan. So, while aging is a problem we have failed to solve for thousands of years, the tools to solve it have finally been discovered and their capabilities are improving exponentially. This is why we can finally be optimistic about the future of anti-aging.
Comentários